TeraHertz Spectroscopy
A Powerful Tool for Material Analysis
Terahertz (THz) spectroscopy offers a unique method for investigating the dynamics of materials across various states. Historically, limitations in source power and detection sensitivity restricted its applications. However, advancements in laser technology have opened doors to powerful and versatile THz systems, including our Time-Domain Spectroscopy (THz-TDS) setup.
Our research leverages the capabilities of THz-TDS setup utilizing a femtosecond laser as its core, providing short pulses of light. This light interacts with either non-linear optical crystals or photoconductive antennas, generating THz pulses that probe the material under study. The modified THz pulse then interacts with a detector operating on a similar principle as the emitter, responding only when triggered by the laser pulse. By employing a mechanical delay line, we systematically acquire multiple measurements of the THz electric field (E-field) at different time delays. This data, represented as a curve of E-field versus time delay, reveals valuable insights into the material's structure and dynamics at the picosecond level.
Unlocking Gas-Phase Information with High-Resolution THz Spectroscopy
The terahertz (THz) range stands out for its exceptional selectivity towards organic volatile compounds, even exceeding the capabilities of the well-established infrared fingerprint region ([Smith, 2015]). Recognizing this advantage, our research aims to leverage the full information contained within THz-TDS time traces for gas sensing applications.
A critical initial challenge was to demonstrate that these traces truly capture the high-resolution gas spectral information, characterized by numerous spectral lines. Traditional limitations arose from the reliance on the Fourier transform, impacting overall resolution. To overcome this constraint, we implemented a super-resolution methodology based on a constraint reconstruction approach ([Eliet, 2021]). This approach resulted in a remarkable 30-fold improvement in resolution, conclusively demonstrating that THz-TDS time traces hold the necessary high-resolution information for robust gas sensing.
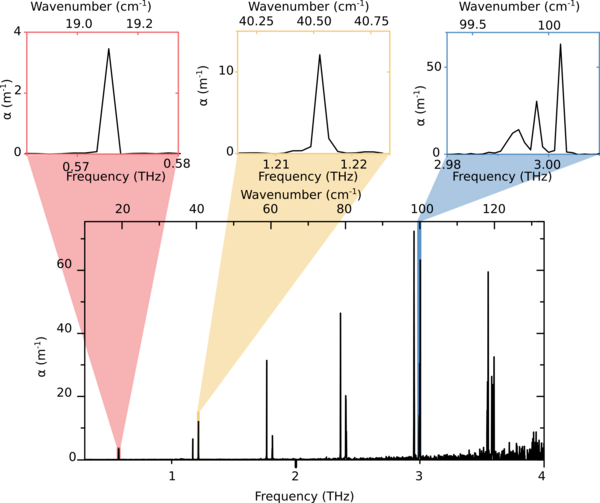
Unveiling the Secrets of Water Dynamics with THz Spectroscopy
Water exhibits unique properties in its liquid state, primarily due to a complex network of hydrogen bonds. These bonds hold these light molecules together, preventing them from transitioning to a gas phase. As with any material, water's dielectric function directly relates to the dynamics of its internal charges. For polar liquids like water, the primary dynamic involves the motion of individual molecules' dipole moments.
Traditionally, the Debye model, developed nearly a century ago, described this low-frequency dynamic ([Debye, 1936]). However, this model rests on several key assumptions: negligible interaction between water molecules, low excitation frequencies compared to their rotations, and the omission of the hydrogen bond network's influence. In the THz range, all these assumptions break down, making THz water spectroscopy both scientifically intriguing and potentially applicable to molecular biology through the investigation of picosecond molecular dynamics.
Our current research focuses on designing experiments capable of delivering highly precise and reproducible measurements of the THz water spectrum across a wide temperature range. Additionally, we collaborate with experts to build analytical models that can better explain water's motion at this specific timescale.
Solid Phase: Unraveling Material Structure with THz Spectroscopy
THz spectroscopy offers a valuable tool for investigating the dynamics of solid materials at the picosecond timescale, analogous to its applications in liquids and gases. In organic solids, this translates to probing collective vibrations that encompass the entire structure. As demonstrated by the historical debate between Euler, d'Alembert, and Bernoulli, a link exists between object size and resonant frequency, evident in musical instruments. THz spectroscopy leverages this principle at the nanoscale, delving deeper than conventional infrared spectroscopy, which primarily probes on individual or few molecular bonds.
Our recent research on lactose crystals exemplifies this capability. Despite possessing the same molecular composition (lactose), we observed distinct THz spectra for different crystal phases. This finding underscores how, nanoscale variations in the crystal structure impact the material's vibrational response at the THz range, reflecting vibrations extending beyond the single molecule and spanning the nanoscale.